Host cell proteins (HCPs) are process-related impurities produced by host cells and are typically present at low levels in recombinant biopharmaceutical products. Enzyme-linked immunosorbent assay (ELISA) has traditionally been used to monitor the total content of HCP in the production of therapeutic protein drugs. Now, with the continuous improvement and upgrading of technology, liquid chromatography-mass spectrometry (LC-MS) is also increasingly used for the qualitative or quantitative analysis of HCP in the purification process.
However, it may be difficult to detect HCP directly by LC-MS because the concentration difference between HCP and therapeutic proteins in solution may exceed 5 orders of magnitude. Combining molecular weight cut-off (MWCO) and proteomics techniques can overcome this difficulty and effectively improve the efficiency of HCP identification and detection. The interaction between HCPs and therapeutic proteins is first removed by anionic surfactants, so that HCPs can be enriched using a molecular weight cut-off (MWCO) ultrafiltration step, and then identified by a "shotgun" quantitative proteomics technique and analysis of HCP in therapeutic protein drugs. This strategy reduces the concentration span of HCP by a factor of 1000, allowing the detection of HCP down to 1 ppm and/or molecular weights below 20 kDa, and effectively eliminates the interference of target proteins and improves the sensitivity of HCP identification and quantitative analysis.
HCP in therapeutic protein processes and its effects
In the production process of therapeutic proteins based on various animal cell culture , all impurities need to be reduced to acceptable levels in order to improve product purity and meet the needs of clinical research. Among them, host cell protein (HCP) is an important impurity. Even low levels of total HCP impurities can adversely affect the properties of the drug. Some of these HCPs may elicit an immune response even at microinjection, and some may even be biologically active or toxic themselves, potentially altering the binding affinity and potency of the drug. In addition, some HCPs may have enzymatic activity that may lead to degradation of the target product. Also, some HCPs may co-purify with the desired product, making it difficult to remove. Therefore, HCPs in products need to be monitored and controlled to support risk assessment. In general, regulatory agencies require HCP levels in the final drug substance to be less than 100 ppm.
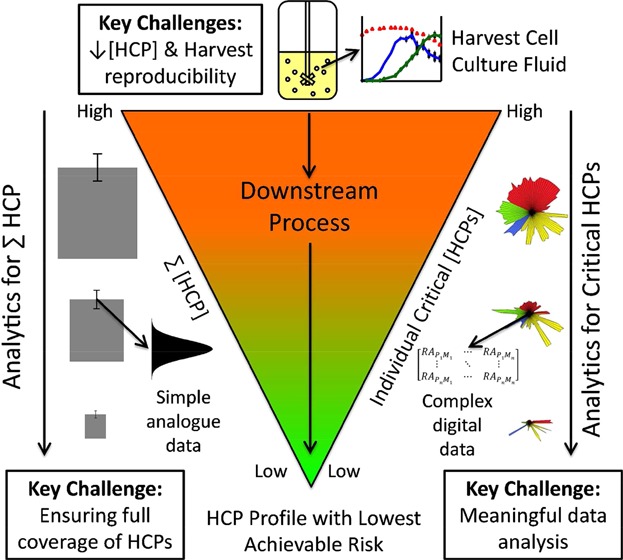
Figure 1. HCP in the biopharmaceutical process
HCP and immunogenicity
The immunogenicity of therapeutic proteins is influenced by four main categories of factors, including: product-related factors, process-related factors, therapeutic procedure-related factors, and patient-related factors. Among them, HCP is an important category of process-related factors. HCP is a heterologous protein for patients, and most heterologous proteins are likely to be immunogenic, so even low concentrations of HCP may trigger an adverse immune response. For example, HCP may cause the production of anti-drug antibodies (ADA) in patients, which may lead to an immune response to the therapeutic protein. ADA may also alter the biological distribution, pharmacokinetics, and biological activity of the therapeutic drug, among other properties. Factors that affect the immunogenicity of HCP include route of administration, dose of HCP, and the number and frequency of repeated administration. With the continuous development of various in vitro and electronic models, it is now possible to predict the immunogenicity of HCP impurities based on their amino acid sequences. However, these models have limited experience in clinical trials and their correlation with clinical outcomes has yet to be investigated in more depth, thus the immunogenicity of HCP may be overestimated or underestimated, so these models should be used with caution in risk assessment.
HCP and Target Protein Degradation
The HCP of the host cell also contains some proteolytically active enzymes, which can fragment the target protein, resulting in a decrease in the total product. Host cell proteases play critical roles in catalysis, metabolic pathways, and turnover of extracellular waste, but when present in the cell culture medium, proteases may lead to product breakdown. This phenomenon occurs in serum-free media, where proteolytic degradation occurs due to the lack of serum protease inhibitors in chemically defined media. During cell culture, proteases can be secreted into the medium or released during cell lysis, thereby accelerating the cleavage of the protein of interest. In addition, glycosidases can trim oligosaccharide chains attached to target protein molecules, resulting in less mature glycan structures, which ultimately affect the pharmacokinetics and pharmacodynamics of glycoproteins.
HCP and aggregation of target proteins
In addition to degradation and fragmentation of target proteins, HCP can also lead to protein aggregation. HCP can alter the structure of proteins, resulting in the formation of soluble aggregates such as dimers of small size, or insoluble visible particles of various larger sizes. Smaller aggregates may trigger immunogenic reactions, and larger particles may lead to various adverse reactions such as immunogenicity.
Binding HCP (BCP) is one of the most abundant proteins in the host proteome and is essential for the correct folding of proteins. However, during high level expression of recombinant protein molecules, upregulation of these Binding HCP expression can exacerbate intracellular aggregation of the target protein, especially if the recombinant protein molecule is incompletely or incorrectly folded leading to aggregation. In large-scale production, natural and correctly folded proteins may interact with Binding HCP to form unnatural aggregates. Binding HCP released during cell lysis interacts with incompletely or incorrectly folded protein molecules in the cell culture medium, resulting in the formation of aggregates through hydrophobic interactions and disulfide bonds. In addition, the β-folded structure of the target protein further promotes its non-specific interaction with HCP, which in turn accelerates the aggregation process.
At different stages in the bioproduction process, protein aggregation may involve HCPs of different properties. In addition to being affected by Binding HCP, protein aggregation may also be associated with a series of proteolytic enzymes. For example, cathepsin D can cleave mAb fragments from intact mAb molecules to form visible particles. Similarly, carboxypeptidase cleaves the C-terminal lysine of mAbs to generate mAb fragments with different charge distributions, and the uneven distribution of surface charge on the protein can destabilize the molecule, leading to aggregation.
In the formulation of therapeutic proteins, additives are usually introduced to improve the stability of the formulation. However, the degradation products of some additive molecules themselves may destabilize the target protein molecules, resulting in protein aggregation. For example, a small amount of triacylglycerol lipase can hydrolyze the ester bond of the surfactant polysorbate 80 (PS80) in the formulation to generate fatty acid and PS85. These two degradation products may combine with the target protein molecule through hydrophobic interaction to form visible particles.
HCP causes Protein-A dissolution and column contamination
There are also catalytically active substances such as metalloproteinases in HCP, which may lead to the dissolution of Protein-A in Protein-A chromatography. The dissolved Protein-A fragments are immunogenic, so they also need to be closely monitored, and the final drug formulation must contain less than 10 ppm of Protein-A. Removal of protein A fragments is challenging and often requires additional purification steps, which increase downstream burden and cost. In addition, as the purification cycle increases, HCPs can accumulate on the surface of the Protein A resin, thereby contaminating the chromatographic resin, resulting in reduced column performance and shortened lifetime.
References
- A. Eon‐Duval, H. Broly and R. Gleixner, Biotechnology progress, 2012, 28, 608-622.
- A. Rosenberg and A. Worobec, 2005.
- A. S. Rosenberg, The AAPS journal, 2006, 8, E501-E507.
- B. Sharma, Biotechnology advances, 2007, 25, 325-331.
- C. Bailey‐Kellogg, A. H. Gutiérrez, L. Moise, F. Terry, W. D. Martin and A. S. De Groot, Biotechnology and bioengineering, 2014, 111, 2170-2182.
- C. H. Goey, S. Alhuthali and C. Kontoravdi, Biotechnology advances, 2018, 36, 1223-1237.
- C. L. Z. de Zafra, V. Quarmby, K. Francissen, M. Vanderlaan and J. Zhu-Shimoni, Biotechnology and bioengineering, 2015, 112, 2284-2291.
- D. G. Bracewell and C. M. Smales, Bioanalysis, 2013, 5, 123-126.
- D. G. Bracewell, R. Francis and C. M. Smales, Biotechnology and bioengineering, 2015, 112, 1727-1737.
Related Service
Related Resource
How to Analysis Host Cell Proteins (HCPs)