In the processes of protein production, transport, and storage, various factors may lead to protein aggregation. A thorough exploration of protein aggregation phenomena has revealed multiple pathways and diverse influencing factors, including physicochemical conditions, translational modifications, and protein structure. Considering the significant impact of protein aggregation on protein activity and homogeneity, delving into the pathways of protein aggregation and investigating methods to control aggregation holds paramount significance in obtaining homogeneous proteins.
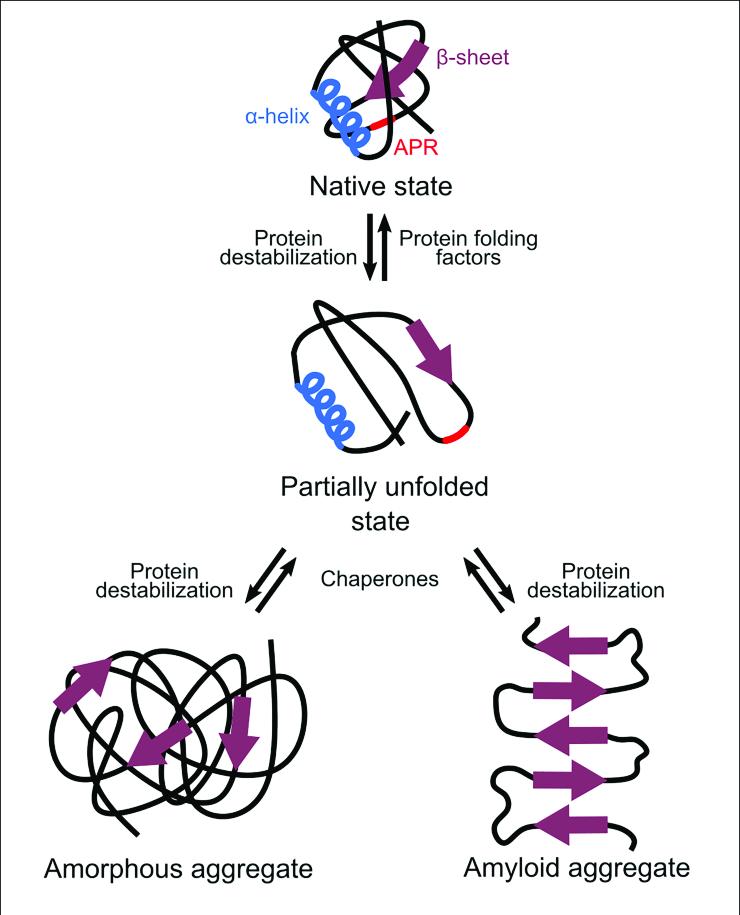
Impact of Protein Aggregation on Protein Pharmaceuticals
Protein aggregation stands as a significant challenge in the research, development, and commercialization of protein-based drugs. Throughout the production, storage, and application of pharmaceuticals, protein aggregation is a phenomenon that occurs, exerting a profound influence on the quality and efficacy of these drugs.
I. Impact on the Production Process of Protein Pharmaceuticals
Reduced Yield in Production:
Protein aggregation leads to a decrease in the yield of proteins during the production process. Interactions between protein molecules during production may induce aggregation, diminishing the content of active components and subsequently affecting the overall drug yield.
Negative Influence on Product Quality and Efficacy:
Protein aggregation significantly impairs the quality and efficacy of the final product. Altered molecular structures resulting from aggregation commonly weaken the biological activity of proteins, leading to a reduction in therapeutic effectiveness.
Potential Immunogenic Risks of Protein Aggregates:
Aggregated protein entities may pose a potential risk of enhanced immunogenicity. Proteins undergoing aggregation exhibit heightened immunogenic properties, increasing the likelihood of triggering immune responses within the body and thereby impacting the safety of the pharmaceutical product.
II. Impact of Protein Aggregation on the Storage and Utilization of Protein Pharmaceuticals
Stringent Storage Conditions:
Protein pharmaceuticals face demanding storage conditions where environmental factors such as temperature and humidity may induce protein aggregation. The high requirements for storage conditions increase the cost and complexity of pharmaceutical storage.
Reduced Drug Stability:
Protein aggregation adversely affects the stability of pharmaceuticals, making them prone to degradation and inactivation during storage and usage.
Clinical Efficacy Instability:
During the therapeutic process within a patient's body, protein aggregation may lead to a weakening of the biological activity of drug molecules, causing instability in clinical efficacy and, in some cases, generating side effects.
In addressing the impact of protein aggregation on protein pharmaceuticals, researchers should thoroughly consider mitigating the risk of protein aggregation in the drug design and production processes. Measures such as optimizing production processes, adjusting drug formulations, and improving storage conditions hold the potential to alleviate the influence of protein aggregation on the quality and efficacy of protein pharmaceuticals. Simultaneously, advancing research on protein pharmaceuticals and gaining insights into the mechanisms of protein aggregation contribute to the development of safer and more effective protein-based drugs.
Select Service
Factors Affecting Protein Aggregation
Different factors exert distinct effects on protein aggregation through diverse mechanisms. Heat induction and changes in protein concentration enhance protein aggregation tendencies through covalent bonding and electrostatic forces. Additionally, the surfactant dodecylphosphocholine interacts with the polar surface of the membrane protein PagP, mediating nonspecific aggregation. pH fluctuations alter the degree of dissociation of acidic and basic amino acids within protein molecules, affecting the formation and stability of hydrogen and ionic bonds. This, in turn, induces protein unfolding or partial unfolding, consequently promoting aggregation.
In the exploration of how protein structure influences aggregation, various factors such as specific structures, regions, and modifications have been identified to impact the aggregation process. Examples include the "hotspot" region of Met80 in Cytochrome C, aggregation-prone regions (APRs) in monoclonal antibodies, free heavy chain (HC) regions, the "TIM barrel structure" folding, N-terminal deletions, and free thiol groups. Notably, free thiol groups in proteins are prone to forming covalent-bonded dimers. Cysteine residues play a crucial role in covalent protein aggregation.
Beyond the mentioned structures, amino acid mutations in the primary structure also influence aggregation. For instance, replacing the Phe24 residue in the hydrophobic cavity of insulin dimers with glycine or alanine reduces the stability of insulin dimers. During protein translation, glutathionylation alters the protein's quaternary structure, transforming protein aggregates from decamers to lower-order aggregates. Deamidation decreases aggregates generated through domain swapping in the 3D structure. Proteins with higher translation rates are prone to unfolding reactions, leading to aggregation.
Pathways of Protein Aggregation
The Role of 3D Domain Swapping in Protein Aggregation
3D domain swapping is a distinctive molecular interaction mechanism. Within biological systems, this phenomenon occurs when two or more partially unfolded monomeric proteins exchange structural domains. It is considered a naturally occurring, potentially reversible process prevalent in all proteins. Initially identified in the study of bovine pancreatic ribonuclease, subsequent research has confirmed the universality of this phenomenon. Explicit evidence of 3D domain swapping was established in the investigation of diphtheria toxin dimers.
This domain swapping predominantly takes place at the N-terminus or C-terminus of proteins, although simultaneous swapping at both terminals is also possible. During the exchange, proteins engage in a cooperative interaction involving the main structure, hinge loops, and swapping regions, resulting in the formation of linear or cyclic aggregates.
Several environmental factors have been identified to influence 3D domain swapping and protein aggregation. These factors encompass ethanol treatment, freeze-drying, salt-buffered solutions, pH levels, high protein concentrations, and the charge distribution on the protein surface. It is noteworthy that these environmental factors exert distinct mechanisms on 3D domain swapping, providing a deeper perspective for understanding protein structure and function.
The Role of Salt Bridges in Protein Aggregates
Within the non-covalent driving forces of protein aggregate formation, such as hydrogen bonds, van der Waals forces, ionic interactions, and hydrophobic interactions, salt bridges are considered manifestations of electrostatic attraction between amino acid residues carrying opposite charges, such as Asp, Glu, Tyr, Cys, His, Lys, and Arg. This attraction primarily stems from hydrogen bonding and ionic interactions. Consequently, in proteins where intrachain salt bridges formed by amino acid residues lead to aggregate formation, they are susceptible to pH influence. The involvement of water molecules and the binding of metal ions both impact salt bridges, with interchain salt bridges generally exhibiting higher stability compared to intrachain ones. In certain proteins, salt bridges play a crucial role in maintaining stable conformational states, such as the connections between different subunits and the formation of dimers.
In protein structures, salt bridges not only facilitate the formation of individual interactions but also contribute to intricate interactions between salt bridges at different locations. Salt bridges tend to form readily in intrinsically disordered proteins (IDPs), owing to their rich content of polar amino acid residues and an abundance of residues carrying opposite charges. Additionally, heat-loving proteins, or thermophiles, often exhibit a higher prevalence of salt bridges, contributing significantly to their enhanced thermal stability.
The Role of Oxidative Stress in Protein Aggregation
The influence of oxidative stress on protein aggregation is substantial, manifesting both in vivo and in vitro. In the realm of neuronal cells, mitochondrial dysfunction elevates the generation of reactive oxygen species, consequently stimulating protein oxidation and triggering aggregation. In vitro studies indicate that factors such as light exposure, additives, and freeze-drying processes can generate free radicals or accelerate protein oxidation-induced aggregation. However, some research suggests that the inducing effect of hydroxyl radicals on protein aggregation is minimal, with aggregation primarily attributed to increased surface area resulting from bubble cavitation.
In the realm of mitigating protein aggregation triggered by oxidative stress, investigations have illuminated the efficacy of combining tryptophan, pyrroloquinoline quinone, tyrosine, or their derivatives with other antioxidants or stabilizers. This collaborative application serves as a potent shield, effectively safeguarding therapeutic proteins from aggregation and oxidative damage amidst oxidative stress. Additionally, substances such as ascorbic acid, N-acetyl-L-cysteine, and L-methionine have been identified as pivotal antioxidants, playing a significant role in the protective process for proteins.
Control of Protein Aggregate
Removal or Modification Hotspot
The primary sequence and higher-order structure of proteins exert a decisive influence on their propensity for aggregation. The process of protein aggregation can be decelerated by removing or modifying sequences that are prone to aggregation, commonly referred to as "aggregation hotspots." Achieving this goal involves analyzing both the protein and its hydrophobic surface. Single-point mutations at times prove effective in reducing the degree of protein aggregation. Furthermore, the connection of hydrophilic structures to shield hotspot regions also contributes to diminishing protein aggregation phenomena, employing methods such as PEGylation, glycosylation, among others.
Adjustment of Solution Formulation
Modifying protein structures can induce changes in biological function, and adjustments in protein stability are often made by modifying the formulation to reduce aggregation risks. Such adjustments primarily involve tuning solution pH, ionic strength, and stabilizer concentrations. Some surfactants, like glycerol, can prevent protein aggregation by inhibiting protein unfolding and stabilizing partially unfolded intermediates. Additionally, surfactants such as sodium dodecyl sulfate (SDS), sodium dodecylbenzenesulfonate, and N-alkylpyrrolidone glycosides can reduce protein aggregation at sub-micellar and micellar concentrations. It is noteworthy that SDS, apart from reducing aggregation, may induce protein aggregation, a phenomenon related to solution pH.
Research indicates that anionic and cationic surfactants distribute differently on the protein surface, possibly due to variations in the filling degree of hydrophobic clefts by hydrophilic groups and alkyl chains. Therefore, a comprehensive consideration is necessary when selecting suitable surfactants to suppress aggregation. Additionally, amino acids and other organic compounds (e.g., arginine, polyarginine, L-proline, L-isoleucine, aromatic naphthoquinone-tryptophan hybrid molecules, vitamin K3, etc.) can serve as inhibitors to restrain protein aggregation.
Frequently employed protein stabilizers primarily encompass surfactants that diminish surface aggregation of proteins, such as PS, alkyl glucosides, amino acid derivatives, and more. The mechanism of action for surfactants involves potentially reducing protein self-binding, adsorption, and interactions between proteins and other aggregating substances, thereby mitigating protein aggregation. Additionally, sugars, as another category of protein stabilizers, primarily function by enhancing conformational stability to reduce protein aggregation tendencies. Meanwhile, polyols, amino acids, polyamines, and similar substances have been confirmed to have an effect in diminishing protein aggregation.
Of particular note, arginine stands out as an effective protein stabilizing additive. Its efficacy lies in reducing the hydrophobicity of the protein surface through mechanisms such as electrostatic interactions, hydrophobic interactions, and cation-π interactions, thereby maintaining protein stability and minimizing aggregation phenomena.
Lyophilization
In the strategies for controlling protein aggregation, if effective management proves elusive through formulation adjustments, lyophilization emerges as a viable alternative. However, the lyophilization process itself may induce protein aggregation. Therefore, researching and optimizing the lyophilization process to minimize its inductive impact on protein aggregation is equally crucial.
Reference
- Tyedmers, J., Mogk, A. & Bukau, B. Cellular strategies for controlling protein aggregation. Nat Rev Mol Cell Biol 11, 777–788 (2010).