Protein tertiary structure refers to the relative spatial position of all amino acid residues in the entire peptide chain. A polypeptide chain that already has certain α-helix and/or β-sheet regions is folded into a tightly packed, almost spherical space structure, or called the natural conformation. The formation and stability of tertiary structure mainly rely on hydrophobic bonds, salt bonds, disulfide bonds, hydrogen bonds and so on.
An important feature of the tertiary structure is that the amino acid residues that are far away in the primary structure can be very close in the tertiary structure, and their side chains can interact. While the secondary structure is stabilized by the hydrogen bond formed between the amide and carbonyl group in the skeleton, the tertiary structure is mainly stabilized by non-covalent interactions (mainly hydrophobic interactions) between the side chains of amino acid residues. Disulfide bonds also contribute to the stabilization of the tertiary structure. In the tertiary structure of a protein, the secondary structure regions are connected by fragments.
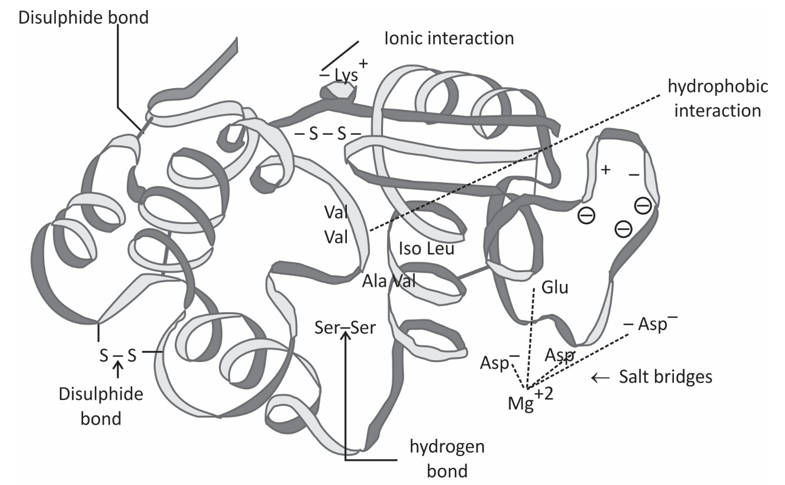
Methods to characterize protein tertiary structure:
The tertiary structure of a protein is the three-dimensional conformation of the protein molecule in its natural folded state. It is formed by further coiling and folding based on the secondary structure. Tertiary structure characterization is vital to the study of protein functional properties. Commonly used methods include X-ray diffraction (XRD), fluorescence spectroscopy (FS), near-ultraviolet circular dichroism (CD) and nuclear magnetic resonance (NMR).
Near UV circular dichroism (CD)
Aromatic amino acid residues, such as tryptophan (Trp), tyrosine (Tyr), phenylalanine (Phe) and disulfide bond in the asymmetric microenvironment, will show CD signal in the near ultraviolet region of 250 ~ 320nm.
The shape and size of the near-ultraviolet CD spectrum are affected by the type of aromatic amino acids in the protein, the environment (including hydrogen bonds, polar groups, and polarizability, etc.) and the spatial location structure.
Near-ultraviolet CD spectroscopy can be used as a sensitive spectroscopic probe, reflecting the disturbance of the microenvironment of Trp, Tyr, Phe and disulfide bonds, and can be used to study the fine changes of the tertiary structure of proteins.
Fluorescence spectroscopy (FS)
When certain substances are irradiated with the light of a certain wavelength, they will emit light with a longer wavelength than the incident light in a short period of time. Such light is called fluorescence. There are tryptophan (Trp), tyrosine (Tyr), phenylalanine (Phe), and other amino acids with a benzene ring or conjugated double bond in protein molecules, which can produce fluorescence under a certain excitation wavelength, so the protein has endogenous fluorescence. Fluorescence spectroscopy can measure the fluorescence intensity of the protein and the reaction system to study the change of the overall spatial conformation of the protein during the denaturation or renaturation process.
NMR technology uses the chemical shifts of different atoms (1H or 13C) to reconstruct the chemical connection between atoms, thereby obtaining structural information of the protein, and then revealing the three-dimensional structure of the protein at the atomic level. NMR can be used to analyze large molecular weight samples without limiting the sample state and does not require crystallization during use. In addition, with its high resolution, it is widely used in the determination of the three-dimensional structure of proteins.