What is a Thiol?
A thiol, in its most fundamental definition, is a chemical compound characterized by the presence of a sulfhydryl group (-SH). This molecular arrangement comprises a sulfur atom bonded to a hydrogen atom. Thiols are often referred to as mercaptans due to their ability to capture (or bind) mercury compounds. The sulfhydryl group is a crucial functional group in organic chemistry, conferring unique properties and reactivities to the molecules that bear it.
Thiols exhibit a distinct odor often described as "skunky" or "rotten egg-like," owing to their presence in compounds such as methanethiol, a common component of the scent of skunks. This characteristic odor, while sometimes unpleasant, serves as a useful indicator for the presence of thiols in various substances.
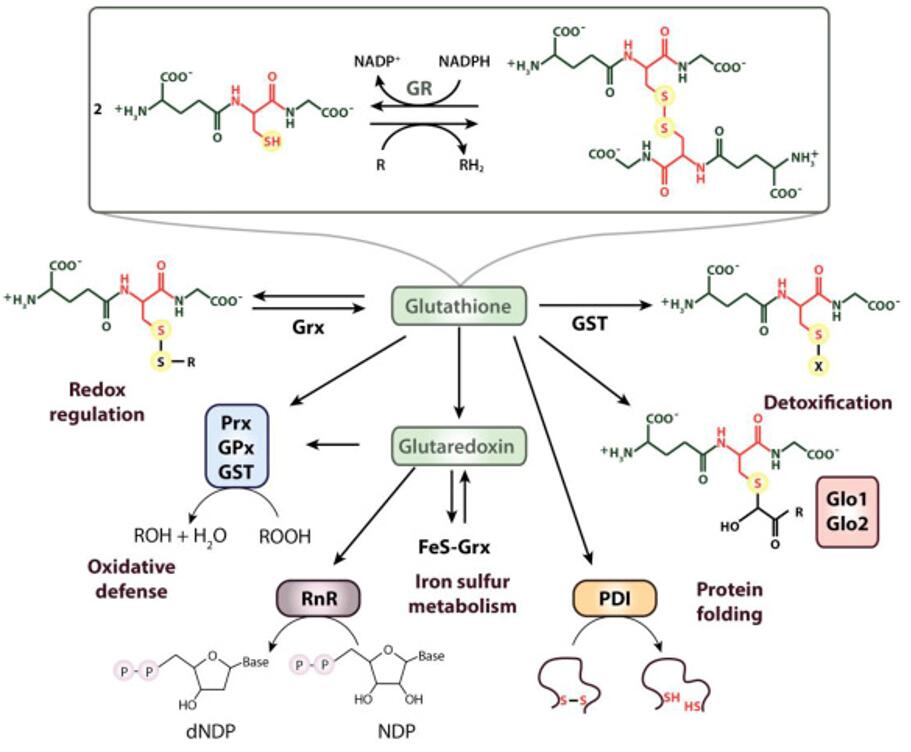
The role of thiols in antioxidant systems (Ulrich et al., 2019)
One prominent example of a thiol compound is cysteine, an amino acid found abundantly in proteins. Cysteine plays a pivotal role in protein structure and function, contributing to the formation of disulfide bonds that stabilize the three-dimensional folding of proteins. Beyond its structural significance, cysteine also participates in redox reactions, acting as a reducing agent due to the presence of its sulfhydryl group.
Thiols encompass a diverse array of compounds found in biological systems, ranging from small molecules like cysteine and glutathione to larger biomolecules such as enzymes and peptides. Their presence and functionality extend throughout various physiological processes, highlighting their importance in maintaining cellular homeostasis and overall organismal health.
Thiol Functions in the Body
Thiols play multifaceted roles within the intricate landscape of the human body, contributing to vital physiological processes essential for health and wellbeing. At the core of their functionality lies the sulfhydryl (-SH) group, which endows them with unique chemical properties that enable interactions critical to biological systems.
One primary function of thiols in the body is their involvement in redox (reduction-oxidation) reactions. The sulfhydryl group readily donates electrons, making thiols potent reducing agents. This ability is exemplified by glutathione, a tripeptide composed of glutamate, cysteine, and glycine, which serves as a principal antioxidant in cells. Glutathione participates in detoxification processes by neutralizing reactive oxygen species (ROS) and free radicals, thereby protecting cellular components from oxidative damage. The thiol group of cysteine within glutathione acts as the active site for electron donation, underscoring its crucial role in maintaining cellular redox balance and combating oxidative stress.
Furthermore, thiols contribute to the structural integrity of proteins through the formation of disulfide bonds. Cysteine residues within polypeptide chains can undergo oxidation to form disulfide bridges (-S-S-), thereby stabilizing protein tertiary and quaternary structures. This process is essential for the proper folding and stability of numerous proteins, including enzymes, hormones, and structural proteins like collagen. Disruption of disulfide bonds can compromise protein function and lead to pathological conditions, highlighting the significance of thiols in protein structure maintenance.
Thiols also serve as coenzymes or cofactors for various enzymatic reactions. Many enzymes require thiol-containing compounds, such as coenzyme A (CoA), for their catalytic activity. CoA plays a pivotal role in cellular metabolism by facilitating the transfer of acyl groups during processes like fatty acid oxidation and the citric acid cycle. Additionally, certain enzymes rely on thiol groups for their catalytic mechanisms, wherein the sulfhydryl moiety acts as a nucleophile or participates in substrate binding.
Beyond their roles in redox chemistry, protein structure, and enzymatic function, thiols contribute to cellular signaling pathways and gene regulation. Sulfhydryl groups can undergo post-translational modifications, such as S-nitrosylation and S-glutathionylation, which modulate protein activity and signaling cascades. Moreover, thiols are involved in the regulation of transcription factors and gene expression through redox-sensitive mechanisms, influencing cellular responses to oxidative stress and environmental stimuli.
Thiol Functional Group: Characteristics and Significance
The thiol functional group, characterized by a sulfur atom bonded to a hydrogen atom (-SH), exhibits distinctive characteristics that confer both chemical reactivity and biological significance. Understanding the properties of this functional group is crucial for elucidating its roles in various molecular contexts and appreciating its significance in biological systems.
One notable characteristic of the thiol group is its nucleophilicity, stemming from the relatively high electron density on the sulfur atom. This electron-rich nature renders thiols potent nucleophiles capable of participating in a wide array of chemical reactions, including nucleophilic substitution, addition, and redox processes. The ability of thiols to donate electrons makes them essential players in redox reactions, where they serve as reducing agents by transferring electrons to other molecules.
Another defining feature of the thiol group is its propensity for forming strong covalent bonds, particularly with metals such as mercury and gold. Thiol-metal interactions, known as coordination bonds or metal-thiolate complexes, are characterized by the binding of the sulfur atom to the metal center, often resulting in stable coordination geometries. This property finds applications in various fields, including coordination chemistry, materials science, and bioinorganic chemistry.
In biological systems, thiols exhibit remarkable versatility, participating in a myriad of cellular processes essential for homeostasis and survival. One key role of thiols is their contribution to antioxidant defense mechanisms, where they scavenge reactive oxygen species (ROS) and protect cells from oxidative damage. Glutathione, a tripeptide containing a thiol group, serves as a primary antioxidant in cells, highlighting the importance of thiols in maintaining cellular redox balance and mitigating oxidative stress.
Furthermore, thiols play crucial roles in protein structure and function through the formation of disulfide bonds. Cysteine residues within polypeptide chains can undergo oxidation to form covalent disulfide bridges, stabilizing protein tertiary and quaternary structures. Disruption of disulfide bonds can lead to protein misfolding and aggregation, contributing to pathological conditions such as neurodegenerative diseases.
Beyond their roles in redox chemistry and protein stabilization, thiols are involved in cellular signaling pathways, enzymatic catalysis, and metal ion homeostasis. Sulfhydryl groups can undergo post-translational modifications, regulating protein activity and cellular responses to environmental stimuli. Additionally, thiols participate in the coordination of metal ions within proteins and metalloenzymes, influencing their catalytic activities and structural properties.
Identification of Thiols
The identification of thiols, crucial for understanding their presence and roles in various chemical and biological systems, relies on a combination of analytical techniques tailored to detect the characteristic sulfhydryl (-SH) functional group. Given the diverse chemical environments in which thiols may be found, ranging from simple small molecules to complex biomolecules, a variety of methods have been developed for their detection and quantification.
One commonly employed technique for thiol identification is spectrophotometry, which relies on the measurement of absorbance or emission of electromagnetic radiation by thiol-containing compounds. Thiol compounds often exhibit distinct absorbance or fluorescence spectra, allowing for their selective detection and quantification in complex mixtures. Additionally, spectrophotometric assays utilizing specific reagents, such as Ellman's reagent (5,5'-dithiobis-(2-nitrobenzoic acid); DTNB), enable the direct quantification of thiols based on the formation of colored or fluorescent products upon reaction with the sulfhydryl group.
Another powerful method for thiol identification is chromatography, which separates complex mixtures based on differences in their chemical properties. High-performance liquid chromatography (HPLC) and gas chromatography (GC) coupled with various detection techniques, such as ultraviolet-visible (UV-Vis) spectroscopy, fluorescence detection, or mass spectrometry (MS), enable the sensitive and selective analysis of thiols in diverse sample matrices. Chromatographic separation facilitates the identification and quantification of individual thiol compounds, even in complex biological samples like tissues or bodily fluids.
Mass spectrometry (MS) represents a versatile tool for thiol identification and characterization, offering high sensitivity and selectivity for the detection of thiol-containing analytes. Techniques such as electrospray ionization (ESI) and matrix-assisted laser desorption/ionization (MALDI) allow for the direct analysis of thiol compounds in biological samples, providing information on their molecular masses, structures, and fragmentation patterns. Tandem mass spectrometry (MS/MS) techniques further enhance the specificity and accuracy of thiol identification by enabling the characterization of thiol-containing peptides and proteins through sequence analysis.
In addition to spectroscopic and chromatographic methods, biochemical assays based on specific chemical reactions with thiols are widely employed for their identification and quantification. For example, the Ellman's assay mentioned earlier exploits the reaction of thiols with DTNB to produce a colored thiolate anion, which can be quantified spectrophotometrically. Similarly, enzymatic assays utilizing thiol-specific enzymes, such as glutathione reductase or thiolase, enable the measurement of thiol concentrations in biological samples based on enzymatic activity.
Overall, the identification of thiols encompasses a diverse array of analytical techniques, each offering unique advantages in terms of sensitivity, selectivity, and applicability to different sample types. By leveraging these methods, researchers can elucidate the presence, abundance, and roles of thiols in various chemical and biological contexts, advancing our understanding of their significance in health, disease, and drug discovery efforts.
Applications of Thiols
The versatility of thiols extends beyond biological systems to diverse industrial applications. In organic synthesis, thiol chemistry enables the formation of carbon-sulfur bonds, essential for the production of pharmaceuticals, agrochemicals, and materials.
Furthermore, thiols serve as crucial components in the development of sensors for detecting heavy metals, gases, and biomolecules. Their selective binding affinity and signal transduction properties make them invaluable tools in environmental monitoring and medical diagnostics.
Reference
Ulrich, Kathrin, and Ursula Jakob. "The role of thiols in antioxidant systems." Free Radical Biology and Medicine 140 (2019): 14-27.