Function of Oxysterols
xysterols, as oxidized derivatives of cholesterol, play multifaceted roles in biological systems, exerting diverse functions that are critical for maintaining cellular homeostasis and regulating physiological processes. Understanding the functions of oxysterols is essential for elucidating their contributions to health and disease. Below, we delve into the intricate functions of oxysterols:
Regulation of Cholesterol Homeostasis:
One of the primary functions of oxysterols is their involvement in the regulation of cholesterol metabolism and homeostasis. Oxysterols act as intermediates in the metabolic pathways that govern cholesterol biosynthesis, transportation, and elimination. They serve as feedback regulators of cholesterol synthesis by modulating the activity of key enzymes involved in cholesterol biosynthesis, such as 3-hydroxy-3-methylglutaryl-coenzyme A reductase (HMGCR).
Facilitation of Cholesterol Efflux:
Oxysterols play a crucial role in promoting cholesterol efflux from cells, particularly from macrophages in arterial walls. By activating specific transporters, such as ATP-binding cassette transporters A1 (ABCA1) and G1 (ABCG1), oxysterols facilitate the efflux of excess cholesterol to high-density lipoproteins (HDL) for transport to the liver for excretion or recycling.
Modulation of Cellular Signaling Pathways:
Beyond their role in cholesterol metabolism, oxysterols serve as signaling molecules that modulate various cellular signaling pathways. They act as ligands for nuclear receptors, including liver X receptors (LXRs) and oxysterol receptors (OxRs), which regulate the expression of genes involved in lipid metabolism, inflammation, and immune responses. Activation of LXRs by oxysterols promotes the transcription of target genes associated with reverse cholesterol transport, thereby influencing cellular cholesterol levels and lipid homeostasis.
Regulation of Inflammation and Immune Responses:
Oxysterols exhibit immunomodulatory properties and play a role in regulating inflammatory and immune responses. They can act as both pro-inflammatory and anti-inflammatory mediators depending on the context and concentration. Oxysterols regulate the expression of inflammatory cytokines, adhesion molecules, and immune cell functions, thereby modulating the inflammatory milieu and immune cell activation in various tissues and organs.
Induction of Apoptosis and Cell Death:
Certain oxysterols have been shown to induce apoptosis and programmed cell death in various cell types. By activating apoptotic signaling pathways and disrupting cellular membranes, oxysterols contribute to the regulation of cell survival and turnover. However, dysregulated accumulation of oxysterols can lead to excessive cell death and tissue damage, contributing to the pathogenesis of certain diseases, such as neurodegenerative disorders and atherosclerosis.
Regulation of Oxidative Stress and Cellular Redox Balance:
Oxysterols possess redox-active properties and can modulate cellular oxidative stress and redox balance. They act as both inducers and targets of oxidative stress, participating in redox reactions that generate reactive oxygen species (ROS) and reactive nitrogen species (RNS). Oxysterols regulate the expression of antioxidant enzymes and redox-sensitive transcription factors, influencing cellular responses to oxidative stress and maintaining redox homeostasis.
Modulation of Membrane Properties and Function:
As amphipathic molecules, oxysterols interact with cellular membranes and modulate their properties and functions. They influence membrane fluidity, permeability, and lipid raft organization, thereby affecting membrane-dependent processes such as signal transduction, membrane trafficking, and ion channel activity. Oxysterols also play a role in lipid raft-mediated endocytosis and intracellular trafficking of cholesterol and lipoproteins.
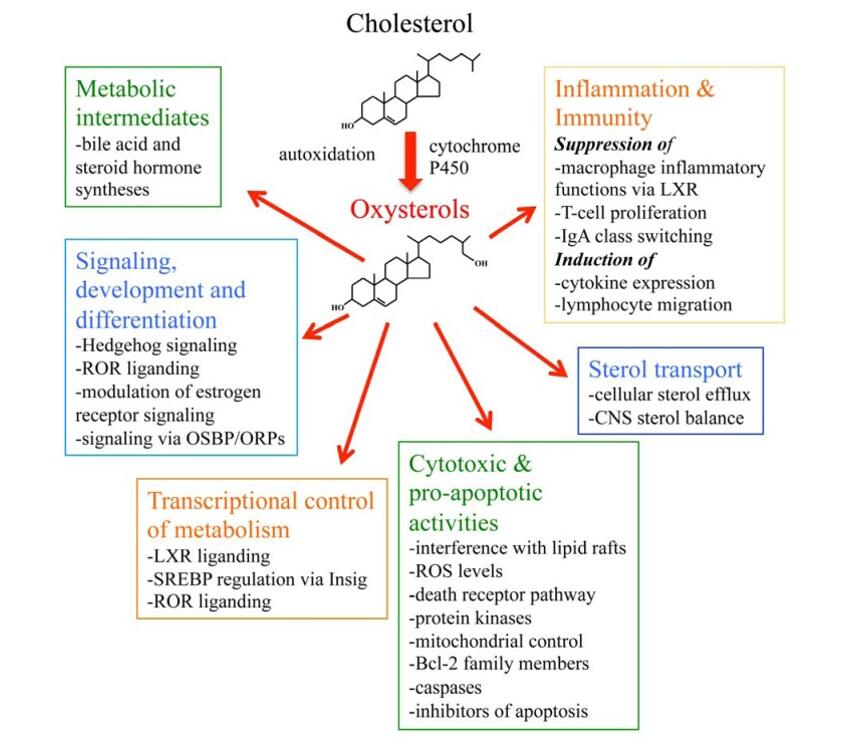
A schematic presentation summarizing the major functions of oxysterols (Olkkonen et al., 2012).
Names and Compounds of Oxysterols
Oxysterols encompass a diverse array of compounds, each distinguished by the position and nature of the oxidized moiety on the cholesterol molecule. Some of the commonly encountered oxysterols include:
- Cholestanetriol: Also known as 5α-cholestan-3β,5,6β-triol, this oxysterol is formed by the oxidation of the 5,6-double bond in cholesterol.
- 7-Ketocholesterol: Formed by the oxidation of the 7-hydroxyl group of cholesterol, 7-ketocholesterol is recognized as a major oxysterol present in oxidized low-density lipoproteins (LDL).
- 24(S)-Hydroxycholesterol: This oxysterol is generated by the hydroxylation of cholesterol at the C-24 position and serves as an endogenous ligand for LXRs.
- 25-Hydroxycholesterol: Arising from the oxidation of cholesterol at the C-25 position, 25-hydroxycholesterol exhibits regulatory effects on cholesterol biosynthesis and cellular cholesterol levels.
- 27-Hydroxycholesterol: As the most abundant oxysterol in circulation, 27-hydroxycholesterol is produced by the oxidation of cholesterol at the C-27 position and plays a critical role in cholesterol metabolism and transport.
What are Oxysterols in Food?
Oxysterols are oxidized derivatives of cholesterol that can be found in various food sources, particularly those containing cholesterol-rich ingredients. The presence of oxysterols in food is primarily attributed to lipid oxidation processes that occur during food processing, storage, and cooking. While oxysterols are not intentionally added to food, their formation is a natural consequence of lipid oxidation, which can occur through exposure to oxygen, heat, light, and enzymatic activity. Below are some examples of oxysterols in food:
Animal Products:
- Meat: Beef, pork, lamb, and other animal meats contain cholesterol, which can undergo oxidation during cooking processes such as grilling, frying, and roasting. As a result, cooked meats may contain various oxysterols derived from cholesterol oxidation.
- Poultry: Chicken, turkey, and other poultry products also contain cholesterol and are susceptible to oxidation during cooking. Oxysterols may form in cooked poultry products as a result of lipid oxidation.
- Dairy Products: Milk, cheese, butter, and other dairy products contain cholesterol, and their lipid content makes them prone to oxidation during storage and processing. Oxysterols may be present in aged cheeses and other dairy products as a result of lipid oxidation over time.
Processed Foods:
- Fried Foods: Deep-fried foods such as French fries, fried chicken, and potato chips are rich in fats and cholesterol and are subjected to high-temperature frying, which promotes lipid oxidation. Oxysterols may be generated during the frying process and contribute to the overall oxidative stability of fried foods.
- Processed Meats: Processed meat products such as sausages, bacon, and hot dogs often contain added fats and undergo processing methods such as curing, smoking, and cooking, which can lead to the formation of oxysterols through lipid oxidation.
Plant-Based Foods:
- Oils and Fats: Plant-based oils and fats, including olive oil, sunflower oil, and soybean oil, contain unsaturated fatty acids that are susceptible to oxidation. Heating oils during cooking processes can promote lipid oxidation and the formation of oxysterols.
- Nuts and Seeds: Nuts and seeds contain fats and may undergo oxidation during storage or processing. Oxysterols may be present in roasted nuts and seeds as a result of lipid oxidation during roasting.
Other Food Sources:
- Eggs: Egg yolks are a significant source of cholesterol, and cooking methods such as frying and boiling can promote cholesterol oxidation and the formation of oxysterols in cooked eggs.
- Seafood: Certain types of seafood, such as shrimp, crab, and fish roe, contain cholesterol and may undergo oxidation during processing or cooking, leading to the formation of oxysterols.
Analytical Techniques for Oxysterol Analysis
Analytical techniques for oxysterol analysis are essential tools for researchers and food safety professionals to accurately detect and quantify oxysterols in various samples, including biological tissues, food products, and environmental samples. These techniques employ sensitive and selective methods to identify and measure the diverse array of oxysterol compounds present in complex matrices.
Select Service Oxysterols Analysis Service https://www.creative-proteomics.com/services/oxysterols-analysis-service.htm |
Gas Chromatography-Mass Spectrometry (GC-MS):
Principle: GC-MS combines gas chromatography (GC) with mass spectrometry (MS) to separate and identify oxysterols based on their retention times and mass spectra.
Workflow: Samples are first derivatized to enhance volatility and thermal stability before injection into the GC system. Oxysterols are separated based on their partitioning between a stationary phase and a carrier gas, followed by detection and identification using mass spectrometry.
Advantages: GC-MS offers high sensitivity, specificity, and resolution for oxysterol analysis. It allows for the simultaneous quantification of multiple oxysterols in complex samples with excellent precision and accuracy.
Limitations: GC-MS requires sample derivatization for enhanced volatility, which can introduce additional steps and potential sources of error. It may also have limitations in analyzing thermally labile oxysterols.
Liquid Chromatography-Mass Spectrometry (LC-MS):
Principle: LC-MS combines liquid chromatography (LC) with mass spectrometry (MS) to separate and detect oxysterols based on their retention times and mass-to-charge ratios.
Workflow: Samples are injected into the LC system, where oxysterols are separated on a chromatographic column before entering the mass spectrometer for detection and identification. Electrospray ionization (ESI) or atmospheric pressure chemical ionization (APCI) is commonly used for ionization in LC-MS.
Advantages: LC-MS offers excellent sensitivity, specificity, and versatility for oxysterol analysis. It allows for the direct analysis of complex samples without the need for extensive sample preparation.
Limitations: LC-MS instrumentation and maintenance can be expensive. Method development and optimization may require expertise in chromatography and mass spectrometry.
High-Performance Liquid Chromatography (HPLC):
Principle: HPLC separates oxysterols based on their interactions with a stationary phase and a mobile phase under high pressure.
Workflow: Samples are injected into the HPLC system, where oxysterols are separated on a chromatographic column using a mobile phase gradient. Detection can be achieved using UV, fluorescence, or electrochemical detectors.
Advantages: HPLC offers high resolution and sensitivity for oxysterol analysis. It allows for the quantification of specific oxysterols in complex samples with good accuracy and precision.
Limitations: HPLC may have limited specificity compared to mass spectrometry-based techniques. It may also require extensive sample preparation and optimization of chromatographic conditions.
Enzyme-Linked Immunosorbent Assay (ELISA):
Principle: ELISA is an immunological method that uses antibodies to detect and quantify oxysterols based on specific antigen-antibody interactions.
Workflow: Samples are immobilized on a solid surface and incubated with oxysterol-specific antibodies. After washing to remove unbound antibodies, a secondary antibody conjugated to an enzyme is added, followed by a substrate to produce a measurable signal.
Advantages: ELISA offers rapid, cost-effective, and high-throughput screening for oxysterols in biological samples. It does not require specialized instrumentation and can be easily automated.
Limitations: ELISA may lack the sensitivity and specificity of chromatographic techniques. Cross-reactivity with structurally similar molecules and interference from sample matrix components can affect assay accuracy.
Reference
Olkkonen, Vesa M., Olivier Béaslas, and Eija Nissilä. "Oxysterols and their cellular effectors." Biomolecules 2.1 (2012): 76-103.