Isoelectric Point Definition
The isoelectric point (pI) of a molecule represents the pH of a solution at which the molecule's surface carries no net charge or a net charge of zero. The net charge of a molecule is typically influenced by the pH of its environment, primarily through the gain or loss of protons (H⁺) leading to the molecule carrying either a positive or negative charge. Fundamentally, when the net charge of a molecule is zero, the molecular structure may experience a diminished stability due to the potential elimination of both intramolecular and intermolecular electrostatic repulsion and attraction forces.
Isoelectric Point of Protein and Antibody
pI denotes the specific pH at which a protein carries, concurrently, an equal number of positive and negative charges under particular electrical potential criteria. In the context of antibodies, these complex protein entities possess a diverse amino acid structure, integrating positively charged residues such as lysine and arginine, while incorporating negatively charged moieties such as aspartate. The charge configuration of these charged amino acids is dynamic and fluctuates in concert with deviations in the pH environment. When an antibody achieves its isoelectric point, a state of equilibrium is attained such that the distribution of positive and negative charges is equalized, thereby conferring a neutral charge state. This pivotal equilibrium state is critically influential for the stability of proteins and their electrophoretic segregation. Consequently, an in-depth comprehension of the isoelectric point of antibodies is of paramount importance for optimizing conditions for electrophoretic separation, facilitating ion exchange chromatography, and for evaluating protein stability and solubility characteristics.
Isoelectric Point Test Methods
pI values are typically determined using gel-based isoelectric focusing (IEF) or capillary isoelectric focusing (cIEF) methods. Additional, albeit less common, techniques include chromatofocusing, discontinuous electrophoresis, ion-exchange chromatography, and isoelectric precipitation. While IEF and cIEF offer relatively precise results and are applicable to protein mixtures due to the separation based on their pI, their implementation demands high concentrations of sample protein, specialized instrumentation, and a variety of assay components, solutions, and accessories, making the process laborious and time-consuming.
Isoelectric Focusing Electrophoresis (IEF)
Principle and Mechanism of IEF
IEF stands as a robust protein separation method hinging on proteins' pI. During IEF, proteins undergo electrophoresis within a pH gradient until they converge at their respective pI, where their net charge becomes zero. This arrest in migration at the pI facilitates their segregation according to differences in pI values. Typically, the pH gradient is fashioned using carrier ampholytes or immobilized pH gradients (IPGs), thereby furnishing a spectrum of pH values within the gel matrix.
Methodology and Experimental Setup
The experimental arrangement for IEF typically comprises a gel matrix, such as polyacrylamide or agarose, imbued with a pH gradient. Proteins are loaded onto one extremity of the gel and exposed to an electric field. As proteins traverse through the gel, they concentrate at positions corresponding to their respective pI, manifesting as discernible bands or spots along the gel length. The pace of protein migration is contingent upon their charge and the pH gradient, facilitating meticulous separation predicated on pI disparities.
Calibration Using Established Standards
Accurate determination of sample protein pI values necessitates calibration against standards with known pI values. These standards, typically encompassing a spectrum of proteins covering a broad pI range, serve as benchmarks for precise pI determination of unidentified proteins. By juxtaposing the migration patterns of sample proteins with those of standards, researchers can extrapolate the pI values of the target molecules.
Protein Analysis Applications
IEF boasts extensive utility in protein analysis, spanning domains like proteomics, clinical diagnostics, and biopharmaceutical development. It enables the dissection and delineation of intricate protein amalgams predicated on their pI values, facilitating the identification of protein isoforms, post-translational modifications, and biomarkers. Furthermore, the amalgamation of IEF with ancillary analytical techniques such as SDS-PAGE or mass spectrometry affords a comprehensive characterization platform for proteins.
Advantages of IEF
IEF presents numerous benefits, encompassing high resolution, sensitivity, and reproducibility in the separation of proteins. This technique facilitates the analysis of proteins across a broad spectrum of pI, rendering it amenable to both qualitative and quantitative applications. However, the efficacy of IEF hinges upon meticulous optimization of experimental parameters, such as ensuring pH gradient stability, proficient sample loading techniques, and employing appropriate detection methods. Furthermore, the indispensable calibration with standard proteins is imperative to ensure the accuracy of pI determination in IEF analysis.
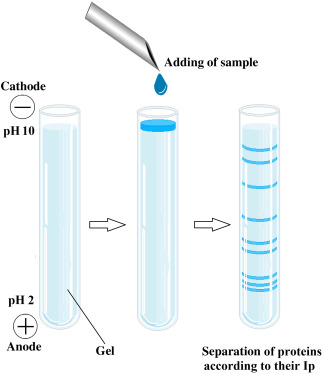
Capillary Isoelectric Focusing (cIEF)
Principle and Instrumentation of cIEF
cIEF emerges as a robust technique for segregating molecules based on their pI. In cIEF, a slender capillary tube is imbued with a pH gradient spanning the desired range, typically from pH 3 to 10. An electric field applied across the capillary propels charged molecules towards their individual pI. The pH gradient within the capillary acts akin to a virtual "sieve," prompting molecules to focus at their pI positions, thus engendering sharp, well-resolved peaks.
Methodology of cIEF
The procedure commences with sample injection into the capillary, followed by mobilization under the influence of an electric field. As the pH gradient stabilizes, proteins migrate towards their respective pI positions, manifesting as distinct peaks along the capillary length. Real-time monitoring techniques, such as UV absorbance or fluorescence, are employed to track the elution of molecules.
Calibration with Marker Proteins
A prevalent strategy in cIEF entails calibrating the pH gradient by employing marker proteins with well-established pI values. These marker proteins, typically comprising a diverse mixture spanning a wide pI spectrum, serve as benchmarks for precise determination of unknown protein pI values. Through meticulous analysis of the migration patterns exhibited by the marker proteins in relation to the sample peaks, researchers can interpolate the pI values of the target molecules with accuracy.
Monitoring Current During Mobilization
An alternative avenue for determining pI in cIEF involves monitoring the electrical current during mobilization. The migration of charged molecules within the capillary elicits electrical signals, which can be correlated with specific pI values. By constructing a calibration graph that correlates current peaks with known pI values, researchers can effectively discern the pI of unknown proteins sans the necessity for internal standards.
Synthetic Peptide Markers
Synthetic peptide markers represent a convenient and reliable method for calibrating pI in cIEF. These peptides, meticulously designed to possess specific pI values, serve as stable and reproducible reference points, facilitating the accurate determination of protein pI values. Integration of synthetic peptide markers into the analytical process enables researchers to validate the pH gradient, thereby ensuring robustness and precision in pI determination.
Applications and Advantages of cIEF
cIEF has garnered extensive utilization across diverse domains, including proteomics, biopharmaceuticals, and clinical diagnostics. Its attributes, including high resolution, sensitivity, and capacity to analyze intricate protein mixtures, render it invaluable for protein characterization, quality control, and biomarker discovery endeavors. Furthermore, cIEF offers notable benefits such as expeditious analysis, minimal sample consumption, and compatibility with automation, rendering it ideally suited for high-throughput applications in both research and industry settings.
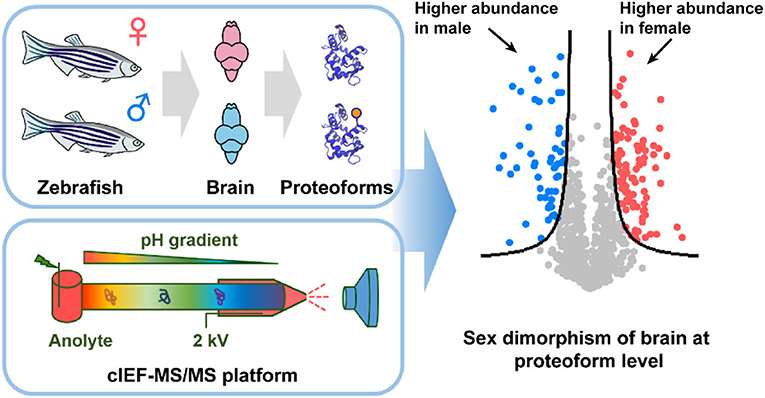
Two-Dimensional Electrophoresis (2D Electrophoresis)
Principles and Workflow of Two-Dimensional Electrophoresis
Two-dimensional electrophoresis (2DE) represents a robust methodology amalgamating two distinct separation techniques: IEF and sodium dodecyl sulfate-polyacrylamide gel electrophoresis (SDS-PAGE), thus facilitating comprehensive protein separation. In the initial phase, proteins undergo separation predicated upon their pI via IEF, resulting in the establishment of a pH gradient within the gel matrix. Subsequently, in the second dimension, proteins are further fractionated based on their molecular weight employing SDS-PAGE. This sequential procedure affords the concurrent assessment of proteins predicated on both their pI and molecular weight, thereby enhancing the depth and precision of protein characterization.
Methodology and Setup of Two-Dimensional Electrophoresis
The implementation of 2DE encompasses a series of procedural intricacies, embracing sample preparation, IEF, equilibration, and SDS-PAGE. Initially, protein specimens undergo solubilization and subsequent loading onto the IEF gel, wherein they migrate contingent upon their respective pI. Following IEF, the focused proteins undergo equilibration to prime them for the subsequent dimension of separation, achieved through SDS-PAGE. Within SDS-PAGE, proteins undergo segregation predicated upon their molecular dimensions, with diminutive proteins traversing the gel matrix at a swifter pace than their larger counterparts. Ultimately, the visualization of protein bands or spots is facilitated via staining methodologies or fluorescent dyes, thereby facilitating the discernment and analysis of individual protein entities.
Applications in Proteomics
The utility of 2DE pervades proteomic investigations, providing a robust platform for the exhaustive scrutiny of intricate protein assemblages. This methodological approach affords the discernment and delineation of protein isoforms, post-translational modifications, and intermolecular interactions. By integrating data pertaining to protein pI and molecular weight, 2DE facilitates the elucidation of protein expression profiles, thereby unearthing potential biomarkers intricately linked with diverse biological phenomena and pathological states. Furthermore, the synergistic amalgamation of 2DE with ancillary analytical modalities, such as mass spectrometry, augments the scope for comprehensive protein identification and quantification.
Advantages and Considerations
The application of 2DE presents numerous advantages, underscored by its high resolution, sensitivity, and capacity for simultaneous analysis across a broad spectrum of proteins. It facilitates the detection of proteins at low abundance levels and affords visualization of intricate protein profiles within biological substrates. Nonetheless, the efficacy of 2DE necessitates meticulous optimization of experimental parameters encompassing pH gradient stability, gel homogeneity, and protein solubilization. Moreover, the intricate nature of protein patterns and the potential for artifact occurrence engender challenges in the realm of data analysis and interpretation. Notwithstanding these considerations, the enduring utility of 2DE endows it as an indispensable tool in the domains of proteomic exploration and biomarker elucidation, serving as a cornerstone in diverse arenas of biological and medical inquiry.
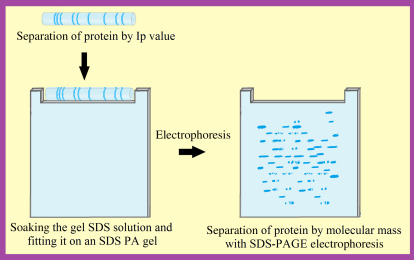
Isoelectric Point Determination Instrument
Principle of Operation
Instruments designed for isoelectric point determination employ IEF technology to ascertain the pI of proteins. This entails the creation of a pH gradient within a gel matrix, often achieved through the inclusion of carrier ampholytes, alongside the application of an electric field to prompt protein migration. As proteins traverse the gel, they gravitate towards their respective pI values. Upon nearing their pI, proteins cease further migration due to attaining a neutral charge state, thus leading to their concentrated localization within the gel.
Instrumentation and Components
Isoelectric point determination instruments are composed of several essential components, comprising a gel electrophoresis chamber, power supply, pH gradient generator, and detection system. The gel electrophoresis chamber serves as the container for the gel matrix, furnishing the substrate for protein separation. The power supply administers the requisite electric field crucial for driving protein migration. Meanwhile, the pH gradient generator orchestrates the establishment of a consistent pH gradient across the gel, typically utilizing carrier ampholytes with predefined ranges of pKa values. Lastly, the detection system enables the visualization and quantification of protein bands or spots residing within the gel.
Workflow and Operation
The operation of an isoelectric point determination instrument encompasses a series of sequential steps. Firstly, the gel matrix is meticulously prepared, and protein samples are meticulously loaded onto the gel substrate. Subsequently, the instrument initiates the creation of a pH gradient across the gel via the pH gradient generator. Guided by the electric field imparted by the power supply, proteins commence migration through the gel matrix towards their individual pI. Upon attaining their pI, proteins halt migration, resulting in the formation of distinct and focused bands or spots within the gel medium. Ultimately, the concentrated proteins are rendered visible and subject to analysis through the application of staining methodologies or fluorescent dyes.
Applications and Significance
Isoelectric point determination instruments hold pivotal significance across diverse domains encompassing research and industrial sectors, spanning proteomics, biotechnology, and pharmaceuticals. Through precise determination of protein pI, these instruments facilitate comprehensive characterization of protein isoforms, discernment of post-translational modifications, and exploration of protein-protein interactions. Moreover, the discernment of pI values is indispensable for refining and optimizing protein purification and separation methodologies. In aggregate, isoelectric point determination instruments furnish invaluable insights into the physicochemical attributes of proteins, thereby streamlining their scrutiny and manipulation across multifaceted applications.
Isoelectric Point Calculation Tools
Online Tools and Software Programs
Numerous computational tools and software applications have emerged to infer the pI of proteins, leveraging their amino acid sequences and associated chemical attributes. These computational resources harness intricate algorithms and expansive databases enriched with structural and functional insights into proteins, facilitating the predictive determination of a protein's pI. Prominent among these resources are online platforms such as ExPASy's Compute pI/Mw tool, ProtParam, and the pI/Mw tool accessible through the SDSC Biology Workbench. Furthermore, standalone software suites such as DNASTAR Lasergene, BioEdit, and ProtParam furnish sophisticated functionalities tailored for pI prediction and comprehensive protein analysis.
Algorithmic Approaches in Isoelectric Point Estimation
The determination of protein pI relies on diverse algorithmic methodologies tailored to analyze protein sequences. These approaches intricately scrutinize the amino acid composition, integrating considerations such as the pKa values of constituent residues, the spatial distribution of charged moieties, and the overall charge profile of the protein. Machine learning algorithms and statistical models, often trained on empirical datasets, further augment these methods, enhancing predictive precision. Beyond furnishing estimated pI values, certain tools offer supplementary insights into protein characteristics, such as molecular weight and charge distribution patterns.
Limitations and Precision
Despite their utility, isoelectric point estimation tools exhibit inherent limitations. Prediction accuracy is contingent upon several variables, including sequence quality, algorithmic intricacy, and the comprehensiveness of associated databases. Moreover, these tools may overlook nuances such as complex protein conformations, post-translational modifications, or interactions with biomolecules, all of which can impact the protein's isoelectric behavior. Consequently, experimental techniques like IEF remain indispensable for attaining precise pI determinations, ensuring rigorous protein characterization.
Complementary Role
Notwithstanding their constraints, isoelectric point estimation tools offer indispensable support within the realm of protein investigation. They afford researchers swift preliminary assessments of pI, streamlining experimental design and facilitating the interpretation of empirical findings. Through synergistic integration with experimental observations, computational predictions enrich our comprehension of protein physicochemical properties, elucidating intricate behaviors across diverse environmental contexts. In sum, these tools constitute integral components of the protein characterization arsenal, fostering advancements in proteomics, bioinformatics, and pharmaceutical research domains.
Other Methods
Less commonly utilized techniques such as chromatofocusing capitalize on the principle of pH gradient separation within chromatography columns, allowing for the isolation of proteins based on their respective isoelectric points. This method offers a heightened degree of separation compared to conventional gel electrophoresis and capillary isoelectric focusing methodologies.
Discontinuous electrophoresis represents another infrequently employed approach, where the pH gradient and electrophoretic processes are segregated, facilitated by a discontinuous pH buffer system. Although less prevalent in practice, this method presents distinct advantages in select research contexts.
Ion-exchange chromatography stands as a method frequently enlisted for isoelectric point determination, leveraging alterations in protein charge properties across varied pH regimes to effectuate separation. Widely embraced in biopartitioning and purification endeavors, this technique demonstrates commendable efficacy in furnishing high-purity protein specimens.
Lastly, the technique of isoelectric precipitation exploits the diminished solubility of proteins proximate to their isoelectric points for separation purposes. Although not commonly invoked, this method can prove efficacious and straightforward under specific circumstances.
Comparison of IEF and cIEF
To provide a comprehensive comparison between IEF and cIEF, we summarize the key features of both techniques in the table below:
Feature | Isoelectric Focusing Electrophoresis (IEF) | Capillary Isoelectric Focusing (cIEF) |
Resolution | High | High |
Sensitivity | High | High |
Sample Handling | Requires specialized equipment and expertise | Requires specialized instrumentation |
Analysis Time | Longer | Shorter |
Sample Consumption | Higher | Lower |
Automation Potential | Limited | High |
Application | Suitable for research laboratories and large-scale protein separations | Ideal for high-throughput analysis and automation in biopharmaceuticals |
In conclusion, both IEF and capillary cIEF stand as potent methodologies, affording high resolution and sensitivity in protein pI determination. Whereas traditional IEF finds its niche in research settings and extensive protein separations, cIEF emerges as the preferred choice for high-throughput analyses and automated processes within the realm of biopharmaceutical development and manufacturing. Researchers are encouraged to judiciously select the most fitting technique according to their experimental requisites and available resources.
Advantages and Disadvantages of Isoelectric Point Test
Advantages | Disadvantages |
Provides crucial information about protein electronegativity | Requires highly purified protein samples |
Aids in optimizing protein purification and stability | Specialized instrument requirements, not suitable for all laboratories |
Used in biopharmaceutical development and quality control | Not sufficiently accurate for complex multi-protein systems |
Applicable for studying protein-protein interactions | Detection requires considerable time, not suitable for rapid analysis |
Utilized in studying protein functional activity and stability | Relies on amino acid sequence information to estimate the isoelectric point |
The detection of antibody isoelectric points stands as a pivotal technique within biological analysis, pivotal in unraveling the intricacies of protein charge distribution. Its significance resonates across diverse domains including protein separation, purification endeavors, stability assessments, and the realm of drug development. Leveraging an array of experimental methodologies and specialized tools, scientists are empowered to meticulously ascertain the isoelectric points of antibodies, thereby enriching our comprehension and harnessing the full potential of these indispensable biomolecules.
Applications of the Isoelectric Point
Determining the isoelectric point of antibodies holds paramount importance across various biological analysis applications. Here are key areas of its application:
Electrophoretic Separation
The precise determination of the pI assumes a pivotal role in the meticulous optimization of protein electrophoresis conditions, particularly in the specialized realm of antibody separation and analysis. Through strategic manipulation of pH parameters guided by the unique pI values of antibodies, researchers can attain an ideal milieu for electrophoretic separation, thereby facilitating the nuanced characterization and quantitative assessment of antibodies within complex biological specimens. This strategic alignment of pH conditions with antibody pI values stands as a cornerstone in ensuring the fidelity and reliability of antibody-related analyses in diverse biological contexts.
Ion Exchange Chromatography
Understanding the isoelectric point of antibodies holds paramount importance in guiding the selection of optimal chromatographic columns and solvent compositions for ion exchange chromatography. Aligning the pH of the buffer system with the specific pI of the target antibody enables the efficient separation and purification of antibodies, thereby laying the groundwork for downstream applications including antibody-based assays and the production of therapeutic antibodies. This strategic alignment of pH conditions with antibody pI values serves as a cornerstone in ensuring the efficacy and success of diverse antibody-related endeavors.
Solubility Control
Comprehending the pI of antibodies is fundamental for managing their solubility across diverse pH environments. In proximity to the pI, antibodies attain electrical neutrality and manifest their minimum solubility, frequently precipitating out of solution. Skillful manipulation of pH in the vicinity of the pI empowers researchers to modulate antibody solubility, facilitating the proficient manipulation and preservation of antibody solutions across a spectrum of biological and biotechnological contexts. This nuanced control over antibody solubility stands as a cornerstone in ensuring the efficacy and reliability of antibody-based methodologies and applications.
Charge State Analysis
Proteins, antibodies among them, exhibit alterations in their charge characteristics in response to pH variations. Understanding the isoelectric point assumes pivotal significance in probing the charge dynamics of antibodies and their interplay with diverse biomolecules. Through systematic manipulation of pH conditions and scrutiny of resultant changes in charge profile, researchers unlock valuable insights into the structural intricacies and functional attributes of antibodies. This comprehensive comprehension not only enriches our understanding of antibody-mediated biological phenomena but also elucidates the underlying mechanisms driving therapeutic efficacy. Such investigations serve as foundational pillars in advancing knowledge across the spectrum of antibody-related research endeavors.
Functional Activity Studies
The functional efficacy of antibodies intricately hinges upon their charge status, a characteristic intricately modulated by the prevailing pH milieu. A nuanced comprehension of the isoelectric point serves as a linchpin for researchers, facilitating the meticulous optimization of antibody functionality through judicious pH selection. This strategic alignment of pH conditions with antibody pI values proves instrumental in enhancing the efficacy and reliability of diverse antibody-centric methodologies, including antibody-based assays, immunoassays, and therapeutic interventions. By upholding antibodies within proximity to their pI, researchers safeguard their functional integrity, thus augmenting their utility across a spectrum of biomedical and biotechnological applications. Such precision in pH management underscores the pivotal role of the isoelectric point in shaping the landscape of antibody-focused research and application endeavors.
Stability Studies
The evaluation of antibody stability across varying pH regimes stands as a cornerstone in ensuring their sustained integrity and efficacy during long-term storage and stability assessments. Armed with insights into the isoelectric point, researchers are equipped to scrutinize the pH-dependent stability landscape of antibodies comprehensively. This informed approach aids in the identification of optimal storage conditions aimed at mitigating degradation and preserving antibody quality over extended durations. By conducting meticulous stability investigations proximal to the pI, researchers safeguard the potency and functionality of antibodies, thereby bolstering their suitability for deployment across a spectrum of diagnostic assays, therapeutic formulations, and diverse biomedical applications. Such strategic endeavors underscore the pivotal role of isoelectric point knowledge in underpinning the reliability and utility of antibody-based methodologies in biomedical research and practice.
Drug Development
In the realm of antibody drug development, a nuanced comprehension of the isoelectric point emerges as pivotal in orchestrating the formulation and storage conditions requisite for therapeutic antibodies. By factoring in the unique pI values of antibodies, researchers can engineer formulations imbued with stability, preserving antibody integrity and efficacy across the gamut of manufacturing, storage, and administration processes. Furthermore, leveraging insights into the isoelectric point informs the judicious selection of buffer systems and excipients, augmenting the stability and shelf-life of antibody drugs. Such meticulous considerations not only safeguard the safety and efficacy of antibody therapeutics but also underscore the imperative of isoelectric point knowledge in shaping the landscape of clinical applications in antibody-based medicine.
References
- Klepárnik K, Slais K, Bocek P. Determination of the isoelectric points of low and high molecular mass ampholytes by capillary electrophoresis. Electrophoresis. 1993
- Audain E, Ramos Y, Hermjakob H, Flower DR, Perez-Riverol Y. Accurate estimation of isoelectric point of protein and peptide based on amino acid sequences. Bioinformatics. 2016
- Righetti PG. Determination of the isoelectric point of proteins by capillary isoelectric focusing. J Chromatogr A. 2004
- Pihlasalo S, Auranen L, Hänninen P, Härmä H. Method for estimation of protein isoelectric point. Anal Chem. 2012