The primary manufacturing approach for antibody drugs primarily involves the expression system of mammalian cells. Within the processes of production, storage, and transportation, the product may undergo changes such as aggregation, degradation, and a host of post-translational modifications. These alterations could result in heterogeneity in molecular size, charge, and glycosylation pattern profiles, thus leading to the generation of associated variants. According to the ICH within the Q6B guidance, these variants are classified into product-related substances and product-related impurities based on their potential impacts on product safety and efficacy. Consequently, the identification, quality research, and quality control of these two categories of substances become critically significant. Assurances of the quality and safety of antibody-based drugs, as well as the protection of patients' welfare, rely fundamentally on these processes.
What is Charge Variant
Studies have found that most modifications can alter the charge of the antibody by changing the number or structure of the charged groups, leading to charge heterogeneity. These proteins, exhibiting charge heterogeneity, are referred to as charge variants.
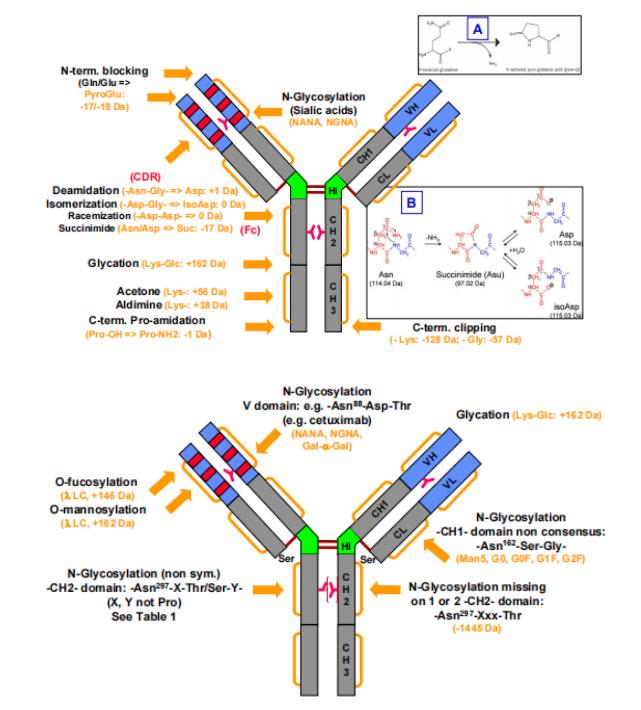
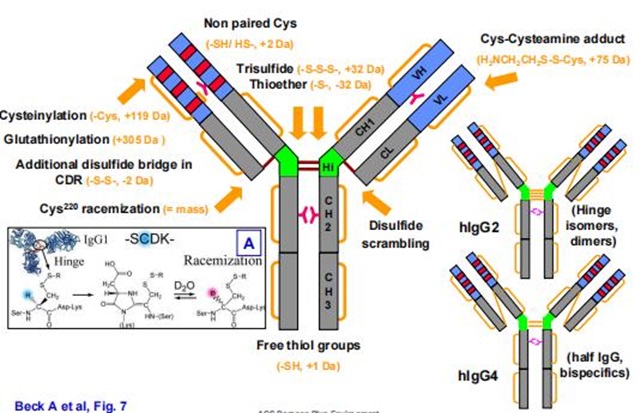
Formation Mechanisms and Impact of Charge Variant
Common Components of the Main Peak
The main peak does not represent an unmodified or undegraded complete antibody molecule. In fact, the main peak is composed of several primary modifications, and common components are illustrated in Table 1:
Table1: Impact of Modifications on Antibody Properties
Number | Modification | Influence |
1 | N-terminal pyroglutamate | No impact |
2 | C-terminal Lys clipping | CDC (Complement-Dependent Cytotoxicity) |
3 | N-Glycosylation (Neutral sugars) | PK/PD, Binding Activity, ADCC, CDC, ADCP, Stability |
Common Components of Acidic Peak
Main causes for the formation of acidic peak primarily involve the introduction of acidic groups on the molecule, the loss of basic groups, and changes in the molecular conformation.
Table 2. Common Constituents of the Acidic Variant (Acidic Peak)
Number | Modification | Influence |
1 | Sialic Acid | PK, ADCC, Immunogenicity |
2 | Deamidation | Binding Activity, Stability |
3 | Succinimide (Asn) | Binding Activity |
4 | Glycation | Aggregation |
5 | O-Glycosylation | Binding Activity, Stability, PK |
6 | Maleuric Acid | Unknown |
7 | Citric Acid | Unknown |
8 | Methylglyoxal | Unknown |
9 | High Mannose | Binding Activity, ADCC, CDC, PK/PD |
10 | Cysteinylation | Stability |
11 | Glutathionylation | Stability, Biological Activity |
12 | Reduced Disulfide Bonds | Stability |
13 | Disulfide Bond Scramble | Biological Activity, Stability |
14 | Trisulfide Bonds | No impact |
15 | Thioether Linkage | Unknown |
Common Components of Basic Variant (Basic Peak)
The formation of basic Variant is principally attributed to the introduction of basic functional groups onto the molecule, the incomplete excision of these groups, and changes in molecular conformation. The common components of the basic isomer, or the "basic peak," are outlined in Table 3.
Table 3: Common Components of the Basic Peak (Basic Variant)
Number | Modification | Influence |
1 | N-terminal Gln/Glu | No impact |
2 | C-terminal Lys | CDC (Complement-Dependent Cytotoxicity) |
3 | Proline Amidation | No impact |
4 | Succinimide (Asp) | Binding Activity |
5 | Mutation form Ser to Arg | Unknown |
6 | Aglycosylation (N-linked) | PK, ADCC, CDC, Stability |
7 | Signal Peptide (Val-His-Ser) (VHS) | Unknown |
Other Modifications
There exists a multitude of modifications that cause variable changes in charge due to aggregation or degradation. These alterations largely depend on specific circumstances, as indicated in Table 4.
Table 4: Other Modifications
Number | Modification | Influence |
1 | Oxidation | Stability, Binding Activity, ADCC, ADCP, PK |
2 | Fragmentation | Biological Activity, PK, Immunogenicity |
3 | Aggregation | Biological Activity, Immunogenicity |
4 | Signal Peptide | Unknown |
5 | Isomerization | Binding Activity |
6 | Sequence Variant | Unknown |
Methods for Charge Variant Detection
Analyzing charge Variant involves segregating and detecting antibodies based on their distinct charges. Current methodologies rely primarily on ion exchange chromatography (IEX), capillary zone electrophoresis (CZE), capillary isoelectric focusing (cIEF), and imaging capillary isoelectric focusing (iCIEF). In chromatographic analysis, acidic Variant, or acidic peaks, have lower pI values than the main substance (Main peak), while basic Variant or basic peaks, possess higher pI values. The ordering of acidic and basic peaks can vary due to different evaluation approaches. For instance, when employing IEX for segregation, acidic Variant are eluted before the main substance, while basic Variant follow after. However, when implementing the CZE methodology, this order reverses.
Characterization Methods for Charge Variants
As previously mentioned, chromatographic and electrophoretic procedures are commonly employed in the separation of charge Variants. The meticulous characterization of these Variants equally presents a challenge.
1. Direct Analysis
1.1 Direct Analysis through IEX-MS
Ion Exchange Chromatography (IEX) is a traditional method for characterizing charge Variants as it separates by leveraging the differences in the surface charges of antibody Variants upon contact with the stationary chromatographic phase. For separating these Variants, methods involving salt concentration gradient, pH gradient, or a combination of both can be employed. However, conventional methods often require high salt concentrations, rendering it incompatible with Mass Spectrometry (MS). Consequently, it cannot be directly applied for charge Variants characterization. To circumvent this issue, volatile salts (such as ammonium salts) can be used to separate in the liquid phase while maintaining the compatibility with MS for detection and identification.
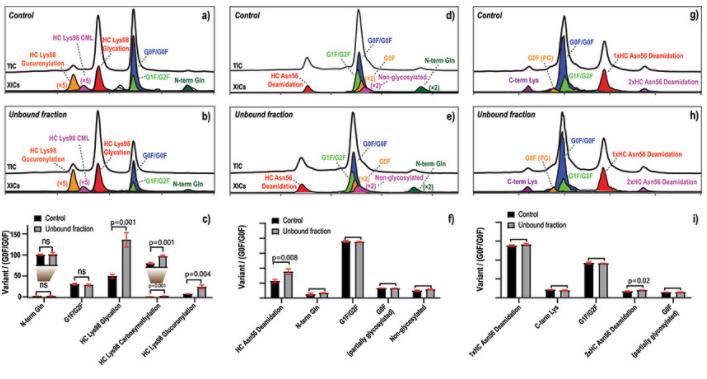
1.2 Direct Analysis through CE-MS
In recent years, capillary electrophoresis and mass spectrometry techniques have realized direct mass spectrometric detection following the separation of antibodies by CE as illustrated in the figure below:
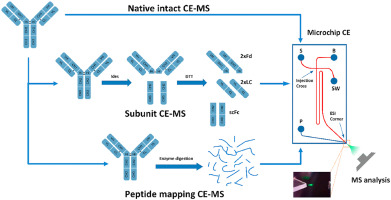
After stringent validation, both these methods have successfully separated charged variants while simultaneously detecting their molecular weights, permitting accurate differentiation of molecular weight discrepancies among various variants. For instance, a molecular weight difference of 128Da signals the variation of lysine. Furthermore, CE-MS technology can also provide precise identification of peptides, even quantifying minor modifications. Yet, these methods bear certain limitations. Both, IEX-MS and CE-MS can only differentiate Variants on the basis of their complete molecular weight or subunit molecular weight and thus may generate significant errors in qualitative analysis. Secondly, these methods require small sample sizes during sample processing, and the online separation enrichment for other type of detection is difficult to achieve. Finally, for samples with high glycosylation, the complexity of glycosylation modification may increase the difficulty of qualitative analysis, affecting the degree of separation and the intensity of mass spectrometric response.
Characterization Following Separation And Collection
Due to the limitations in direct analysis methods, characterization following separation and collection is usually undertaken.
1. Ion Exchange (IEX) Separation and Collection
Antibody charge variants are separated by the IEX method, where samples are collected based on their elution timeframes. These samples are then subjected to a concentration step in preparation for subsequent in-depth characterization.
The scale of separation achieved through the IEX method can be facilely enlarged to procure sufficient sample quantities for mass spectrometry analyses and activity analysis. However, this method offers limited resolving power, impeding the precise separation and collection of each isoform peak. Moreover, the enrichment process requires substantial time and effort.
2. Displacement Chromatography Separation Collection
Displacement chromatography operates as a nonlinear chromatographic technique wherein the introduction of a displacer with a strong affinity for the resin occurs post-sample injection into the chromatography column. This displacer effectively supersedes solute molecules adhered to the surface of the stationary phase. Under the propulsion of the displacer, individual fractions in the sample lineup in a distinct order, forming a series of closely connected bands predicated on the strength of their interactions with the stationary phase. These bands then elute from the column, effectuating an efficacious separation of charge variants.
Upon the comparison with IEX separation and collection methods, the displacement chromatography technique has reflected significant advantages. Specifically, this method boasts substantial sample input, high yield, and paramount resolution. Furthermore, during the process of separation, the components can achieve self-concentration, thereby greatly reducing operational time and labor intensity.
However, an element of caution must be exercised as the displacement chromatography method depends on specific displacers, such as the commercially available cation exchanger Sachem Expell SP1. These displacers not only carry a high cost but also entail a relatively complex selection process. More importantly, a small amount of the displacer can get eluted together with the alkaline variants, which might adversely affect subsequent cell activity analysis and animal testing.
3. Free Flow Electrophoresis (FFE) Separation and Collection
In the FFE process, protein samples are sequentially introduced into the separation chamber and advanced along the length of the chamber under the impact of fluid dynamics. An electric field is applied perpendicular to the direction of fluid flow during the process. The zwitterions present in the separation buffer generate a pH gradient, thereby enabling isoelectric focusing separation based upon the isoelectric point (pI) of charge variants.
Subsequently, the focused variants are expelled from the separation chamber and collected as separate fractions, enabling characterization analyses of different charge variants.
4. Hydrolysis
Notwithstanding the fact that fraction collection is the primary step in a comprehensive analysis of antibody charge variants, before carrying out fraction collection, a proteolytic enzyme can be used to quantify the impact of specific modifications on the charge variants. For instance, the specific contributions of sialic acid to acidic variants can be assessed using sialidase, while the CPB enzyme can determine the extent of lysine contributions to the basic variants.
Impact of Charge variants on Antibody Functionality
The generation of charge variants can significantly influence the pharmacokinetics of antibody drugs. The impact of charge variants on the pharmacokinetics of antibodies has been extensively investigated and widely acknowledged within the industry. Despite the extensive research, the effects of antibody charge variations on their pharmacodynamics appear to be less pronounced. A comprehensive evaluation conducted by industry experts on commercially available antibodies and preclinical antibody drugs regarding the impact of charge alterations on efficacy and pharmacokinetics yielded the following insights:
When the magnitude of charge variation exceeds one pH unit, it significantly affects the tissue distribution and pharmacokinetics of the drug.
An increase in positive charge variation in antibodies promotes drug retention in tissues while simultaneously reducing the blood clearance rate.
Conversely, a decrease in positive charge variation in antibodies reduces drug retention in tissues and enhances the overall systemic clearance efficiency.
Conclusion
In the context of monoclonal antibody production, it is both unrealistic and unnecessary to aim for complete eradication of acidic and basic variants. Certain modifications may be distinctive to recombinant monoclonal antibodies, while others could be linked to specific host expression systems. The impact these modifications impart on biological functions depends on both their location and their extent. For example, modifications in non-critical regions of the Fc domain, even if extensive, may not necessarily cause a significant effect on binding affinity. Similarly, modifications within the Fab region may not alter Fc-related functionalities. However, evaluating the differences in stability and functionality between acidic and basic variants and the main product is of vital importance. Furthermore, the degree of antibody heterogeneity reflects the stability of the production process, highlighting the fact that the characterization and quality control of charge variants should not be overlooked.
References
- Leslie A. Khawli,1,* Sirj Goswami,1 Ryan Hutchinson,et al. Charge variants in IgG1: Isolation, characterization, in vitro binding properties and pharmacokinetics in rats. mAbs. 2010 Nov-Dec; 2(6): 613–624.
- Alain Beck,*,† Elsa Wagner-Rousset, et al. Characterization of Therapeutic Antibodies and Related Products. Anal. Chem. 2013, 85, 715−736
- Yi Du, Alison Walsh, Robin Ehrick, et al. Chromatographic analysis of the acidic and basic species of recombinant monoclonal antibodies. mAbs. 2012 Sep 1; 4(5): 578–585.
- Qiang Sun , Liang Wang , Na Li , Liming Shi. Characterization and monitoring of charge variants of a recombinant monoclonal antibody using microfluidic capillary electrophoresis-mass spectrometry. Analytical Biochemistry. Volume 625, 15 July 2021, 114214.
- Brian D. Hosken, Charlene Li, et al. Isolation and Characterization of Monoclonal Antibody Charge Variants by Free Flow Isoelectric Focusing. Anal. Chem. 2016, 88, 11, 5662–5669.
- Surbhi Gupta, Wim Jiskoot, et al. Oxidation and Deamidation of Monoclonal Antibody Products:Potential Impact on Stability, Biological Activity, and Efficacy. J Pharm Sci. 2021 Dec 7;S0022-3549(21)00646-8.
- Timothy Kaschak, Daniel Boyd, et al Characterization of the basic charge variants of a human IgG1:Effect of copper concentration in cell culture media. mAbs, 2011; 3:6, 577-583.
- Xiaoying (Nancy) Chen, Mary Nguyen, et al. Charge-based analysis of antibodies with engineered cysteines. mAbs. 2009 Nov-Dec; 1(6): 563–571.
- Samantha Ippoliti, et al. Online IEX-MS of mAb Charge Variants Using a BioResolve SCX mAb column, IonHance CX-MS pH concentrates, and Bioaccord System. Waters application note.
- Suresh Babu, Bangalore. Charge Heterogeneity Analysis of Rituximab Innovator and Biosimilar mAbs. Agilent application note.
- C Andrew Boswell 1, Devin B Tesar, et al.Effects of Charge on Antibody Tissue Distribution and Pharmacokinetics. Bioconjug Chem. 2010 Dec 15;21(12):2153-63
- Zhengqi Zhang et al,. A competitive binding-mass spectrometry strategy for high-throughput evaluation of potential critical quality attributes of therapeutic monoclonal antibodies. MAbs. 2022